Introduction to Transmission through Synapses
Synaptic transmission is the process through which neurons communicate with each other, allowing the brain to send and receive signals (Cotman & McGaugh,1980). At the core of this communication are synapses, the tiny gaps between neurons where information is exchanged. When an electrical signal, known as an action potential, reaches the end of a neuron, it triggers the release of chemical messengers called neurotransmitters. These neurotransmitters travel across the synapse and bind to receptors on the next neuron, either exciting or inhibiting its activity. This process enables neurons to transmit signals throughout the nervous system, controlling everything from basic functions to complex thoughts and behaviors.
Understanding synaptic transmission is crucial in psychology as it helps explain how neurons communicate, impacting cognitive functions, emotions, and behavior. This knowledge aids in comprehending neurological disorders like depression or schizophrenia, where neurotransmitter imbalances occur, and informs treatments that target synaptic processes, such as antidepressants or antipsychotics.
Synapse
The term synapse is first coined by English neurophysiologist Charles Sherrington in 1897, derived from derived from the Greek word – synapsis, meaning “conjunction“.
According to American Psychological Association (APA), “the specialized junction through which neural signals are transmitted from one neuron (the presynaptic neuron) to another (the postsynaptic neuron).”
Synapses are the specialized structures that allow one neuron to influence the electrical and biochemical activity of another neuron (Gabbiani & Cox, 2010).
Synapses are the areas where neurons achieve functional connections, and they are also a key part of information transmission.
Neurotransmitter
According to American Psychological Association (APA), “any of a large number of chemicals that can be released by neurons to mediate transmission of nerve signals across the junctions (synapse) between neurons.”
Structure of Synapses
A typical synapse comprises three main parts: the axon terminal, the postsynaptic membrane, and the synaptic cleft.
1. Axon Terminal
The axon terminal, located at the end of an axon, is the presynaptic component of the synapse. It contains several specialized structures:
- Synaptic vesicles: Round granules filled with neurotransmitters, which are the chemical messengers facilitating neural communication.
- Mitochondria: Organelles responsible for meeting the high energy demands of synaptic transmission.
- Tubules: Structural elements that help maintain the shape of the terminal button.
- Storage granules: Found in some axon terminals, these larger structures house multiple synaptic vesicles.
Dark patches in the presynaptic membrane are primarily protein molecules that act as channels and pumps for neurotransmitter release.
2. Postsynaptic Membrane
The postsynaptic membrane is part of the adjacent dendritic spine and lies across the synaptic cleft from the axon terminal. This membrane contains a specialized patch of dark material, which is rich in protein molecules serving as receptors for neurotransmitters. These receptors are essential for receiving and processing chemical messages.
3. Synaptic Cleft
The synaptic cleft is a minuscule gap separating the presynaptic and postsynaptic membranes. This space ensures that the terminal and the dendrite do not make physical contact, enabling neurotransmitters to diffuse and bind to postsynaptic receptors.
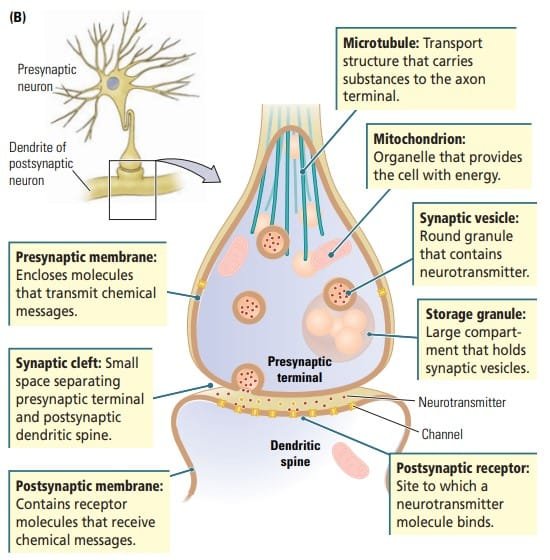
Structure of Synapses. Kolb and Whishaw (2009). Fundamentals of Human Neuropsychology
4. Surrounding Structures
The synapse is embedded within a complex neural microenvironment, often surrounded by:
- Glial cells: Supporting cells that regulate and maintain the synaptic environment.
- Other axons and dendritic processes: Part of the intricate neural network.
- Neighboring synapses: Close clustering of synaptic junctions facilitates neural integration and processing.
The synapse is a highly specialized structure designed for efficient communication between neurons, with its components optimized to support the precise transmission of signals.
Types of Synapses
Structural Types of Synapses
Synapses vary based on the anatomical location of the connection between neurons or other cells. Common types include:
- Axodendritic Synapse : This is the most common type, where the axon terminal of one neuron meets the dendrite or dendritic spine of another neuron (Matthews & Fuchs, 2010). It facilitates the majority of synaptic communications in the brain.
- Axosomatic Synapse : The axon terminal ends directly on the cell body (soma) of another neuron. These synapses are often associated with strong influences on the postsynaptic neuron.
- Axoaxonic Synapse : An axon terminal connects to another axon. Axoaxonic synapses are essential for modulating the activity of the target axon, such as through presynaptic inhibition.
- Axosynaptic Synapse : An axon terminal ends at another axon terminal. This arrangement enables precise control of neurotransmitter release.
- Axoextracellular Synapse : Axon terminals release neurotransmitters into the extracellular fluid without targeting a specific cell. This type supports diffuse signaling.
- Axosecretory Synapse : An axon terminal forms a connection with a capillary and releases neurotransmitters directly into the bloodstream. This is crucial for neuroendocrine signaling.
- Dendrodendritic Synapse : Dendrites communicate with other dendrites without involving axon terminals. These synapses enable localized neural signaling. They are often capable of transmission in either direction (Urban & Castro, 2010).
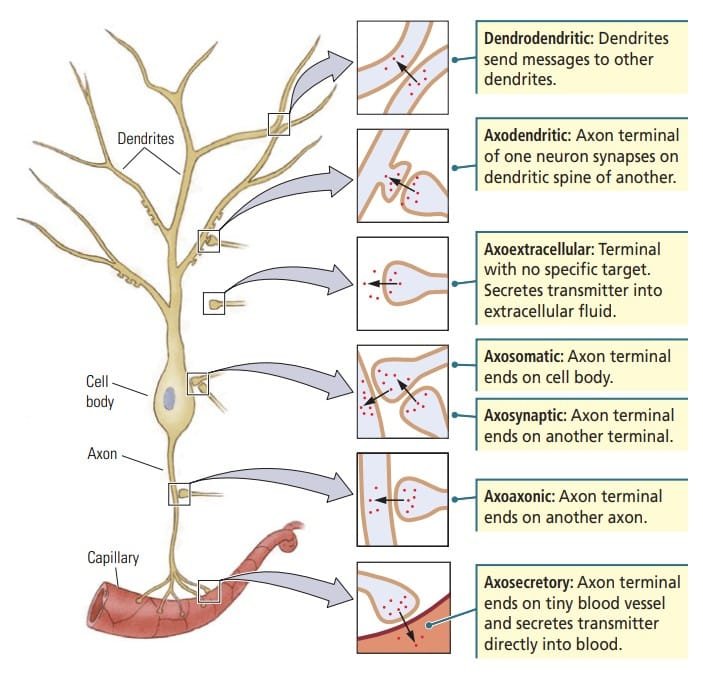
Types of synapses. Kolb and Whishaw (2009). Fundamentals of Human Neuropsychology
Functional Types of Synapses
Synapses also differ in their effects on the postsynaptic neuron, categorized as excitatory or inhibitory:
- Excitatory Synapses (Type I) : Found on dendritic shafts or spines.
- Characterized by:
- Round synaptic vesicles in the presynaptic terminal.
- A dense and wide synaptic cleft.
- Larger active zones where neurotransmitter release occurs.
- Function: Increase the likelihood of an action potential by depolarizing the postsynaptic neuron.
- Characterized by:
- Inhibitory Synapses (Type II) : Typically located on the soma (cell body).
- Characterized by:
- Flattened synaptic vesicles.
- A narrower synaptic cleft.
- Smaller active zones.
- Function: Reduce the probability of an action potential by hyperpolarizing the postsynaptic neuron.
- Characterized by:
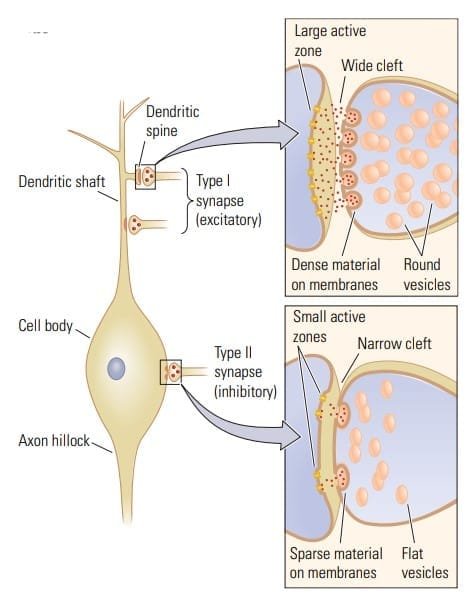
Type I and II synapses. Kolb and Whishaw (2009). Fundamentals of Human Neuropsychology
Excitatory-Inhibitory Integration
The functional division of excitatory and inhibitory synapses reflects the architecture of a neuron:
- Excitatory inputs predominantly reach the neuron via the dendritic tree.
- Inhibitory inputs act closer to the axon hillock, the origin of the action potential, ensuring efficient regulation of neural signaling.
Steps in Synaptic Transmission
Information is transmitted across a synapse in four basic steps. Each step requires a different chemical reaction:
1. During synthesis, either the neurotransmitter is created by the cell’s DNA or its building blocks are imported and stored in the axon terminal.
2. During release, the transmitter is transported to the presynaptic membrane and released in response to an action potential.
3. During receptor action, the transmitter traverses the synaptic cleft and interacts with receptors on the membrane of the target cell.
4. During inactivation, the transmitter either is drawn back into the axon of the presynaptic cell or breaks down in the synaptic cleft. Otherwise, it would continue to work indefinitely.
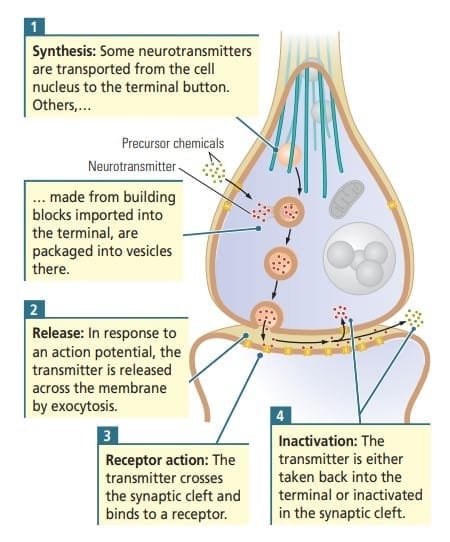
Steps in synaptic transmission. Kolb and Whishaw (2009). Fundamentals of Human Neuropsychology
Read in detail about Neurotransmitters
Step 1 : Synthesis and Storage of Neurotransmitter
Neurotransmission begins with the synthesis and storage of neurotransmitters, the chemical messengers of the nervous system. These molecules can be broadly classified into small-molecule neurotransmitters and neuropeptides, differing in their synthesis pathways, storage, and functions.
- Small-Molecule Neurotransmitters : Synthesized in the cytoplasm of the terminal button and packaged by the Golgi complex. They are stored in clusters near the presynaptic membrane for quick release.
- Neuropeptides : They are assembled as short amino acid chains (3–36 amino acids) in the cell body and packaged in vesicles by the Golgi complex and transported to the terminal at approximately 40 cm/day. Vesicles containing neuropeptides are larger and located farther from the presynaptic membrane.
Neurotransmitters are synthesized via two primary pathways:
- Protein-Derived Neurotransmitters
- Synthesized in the cell body according to instructions in the neuron’s DNA.
- Packaged in membranes by Golgi bodies and transported to the axon terminal via microtubules.
- Messenger RNA may also travel to the axon terminal for localized synthesis.
- Nutrient-Derived Neurotransmitters
- Synthesized in the axon terminal using precursor molecules derived from food.
- Precursor chemicals are absorbed from the blood by transporter proteins in the cell membrane.
- Mitochondria provide the energy required for this synthesis.
These pathways divide neurotransmitters into two categories:
- Quicker-acting small molecules derived from nutrient building blocks.
- Slower-acting proteins derived from DNA.
Storage of neurotransmitters
Once synthesized, neurotransmitters are stored in synaptic vesicles within the axon terminal.
- Storage Granules : Vesicles are clustered within large granules in the terminal.
- Microfilament Attachment : Vesicles are attached to the internal structural filaments of the terminal button.
- Presynaptic Membrane : Vesicles are docked near the presynaptic membrane, ready for immediate release into the synaptic cleft.
Step 2 : Release of Neurotransmitters
Release of neurotransmitter is triggered by the arrival of an action potential at the axon terminal. The axon terminal is rich in voltage-sensitive calcium (Ca²⁺) channels, which play a critical role in this process. The surrounding extracellular fluid is also rich in calcium ions, which are essential for neurotransmitter release.
- Action Potential Arrival : When the action potential reaches the axon terminal, it triggers the opening of voltage-sensitive calcium channels embedded in the presynaptic membrane.
- Calcium Ion Influx : The influx of calcium ions (Ca²⁺) from the extracellular fluid into the terminal occurs rapidly. This sudden influx of Ca²⁺ is a key event that facilitates neurotransmitter release.
- Calmodulin and Vesicle Release : Once calcium ions enter the terminal, they bind to a protein called calmodulin, forming a molecular complex. This complex participates in two crucial reactions:
- One reaction causes the release of vesicles that are bound to the presynaptic membrane.
- The other releases vesicles that are bound to filaments within the axon terminal.
- Exocytosis : The vesicles bound to the presynaptic membrane undergo exocytosis, which is the process where their membrane fuses with the presynaptic membrane, allowing the neurotransmitter to be released into the synaptic cleft. This fusion results in the neurotransmitter being emptied into the gap between the two neurons, making it available to bind with receptors on the postsynaptic membrane.
- Vesicle Recycling : After the vesicles release their contents, the vesicle membranes are recycled. The vesicles previously attached to the filaments move towards the presynaptic membrane to replace those that have been released, ensuring a constant supply of neurotransmitter for future signaling.
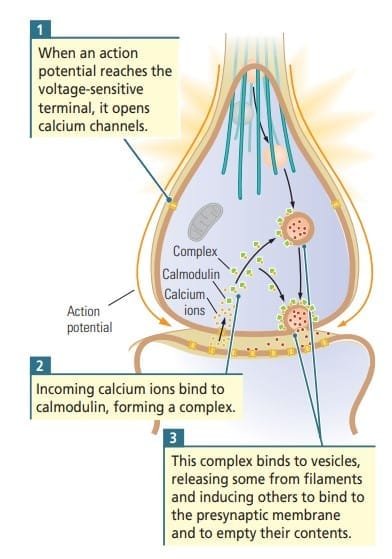
Release of neurotransmitter. Kolb and Whishaw (2009). Fundamentals of Human Neuropsychology
Step 3 : Activation of Receptor Sites
Neurotransmitter Diffusion and Binding
The released neurotransmitter diffuses across the synaptic cleft and binds to specific receptors on the postsynaptic membrane. These receptors are typically proteins that have binding sites for particular neurotransmitters, making each receptor selective for its corresponding ligand.
Types of Effects Based on Neurotransmitter and Receptor Type
The specific effects of neurotransmitter binding depend on the type of neurotransmitter and the receptors on the postsynaptic membrane. These effects may include:
- Depolarization of the postsynaptic membrane, leading to an excitatory action (e.g., EPSPs).
- Hyperpolarization of the postsynaptic membrane, leading to an inhibitory action (e.g., IPSPs).
- Initiation of complex chemical reactions that modulate the excitatory or inhibitory effects or influence other functions of the postsynaptic neuron.
- Creation of new synapses or other long-term changes in the cell.
Autoreceptors: Feedback Mechanism
In addition to binding to postsynaptic receptors, neurotransmitters may also interact with autoreceptors located on the presynaptic membrane. These receptors provide feedback to the cell that released the neurotransmitter, helping regulate neurotransmitter release by:
- Reducing the release when neurotransmitter levels are high.
- Increasing release when neurotransmitter levels are low.
Receptor Subtypes
Initially, it was thought that each neurotransmitter only interacted with one type of receptor, but this is not the case. Most neurotransmitters bind to several different types of receptors known as receptor subtypes. These subtypes are located in different brain areas and typically respond to neurotransmitters in various ways. This enables a single neurotransmitter to transmit different kinds of messages to different regions of the brain.
- Ionotropic Receptors
- Ionotropic receptors are associated with ligand-gated ion channels. When a neurotransmitter binds to these receptors, the ion channel either opens or closes, inducing an immediate postsynaptic potential.
- This can lead to either excitatory postsynaptic potentials (EPSPs) (e.g., opening sodium channels) or inhibitory postsynaptic potentials (IPSPs) (e.g., opening potassium or chloride channels).
- Ionotropic receptors mediate fast and brief responses.
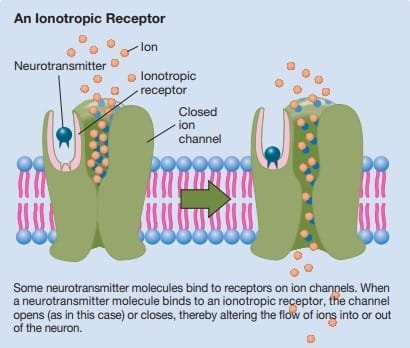
Ionotropic Receptor
J. Pinel & S. Barnes (2018). Biopsychology. 10th ed.
2. Metabotropic Receptors
- Metabotropic receptors are more common and are linked to signal proteins and G proteins inside the cell.
- The binding of a neurotransmitter to a metabotropic receptor leads to activation of the G protein, which can trigger:
- Opening or closing ion channels, leading to either EPSPs or IPSPs.
- Activation of second messengers, which can influence cellular processes such as gene expression and protein synthesis, leading to long-term changes.
- Metabotropic receptors mediate slower, longer-lasting, and more diffuse effects compared to ionotropic receptors.
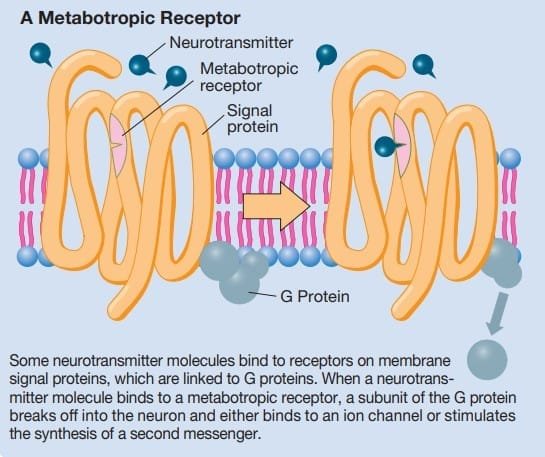
Metabotropic Receptor.
J. Pinel & S. Barnes (2018). Biopsychology. 10th ed.
Step 4 : Deactivation of Neurotransmitters
After neurotransmitters have carried out their function in the synapse, they must be quickly deactivated to prevent ongoing signaling and allow for new messages from the presynaptic neuron. Neurotransmitter deactivation occurs through a variety of processes, ensuring that the signal is terminated and communication within the synapse can be efficiently regulated.
Methods of Neurotransmitter Deactivation
- Diffusion Away from the Synapse : After a neurotransmitter has bound to its receptor and activated the postsynaptic neuron, it may diffuse away from the synaptic cleft, leaving the receptor site and ending its action.
- Enzymatic Degradation
- Some neurotransmitters are broken down directly by enzymes in the synaptic cleft. This process, known as enzymatic degradation, ensures the neurotransmitter is broken into inactive by-products.
- For example, acetylcholine (ACh), a key neurotransmitter in the peripheral nervous system, is broken down by the enzyme acetylcholinesterase into acetate and choline. This degradation prevents prolonged activation of the receptor and terminates the synaptic message.
- Reuptake by Transporters
- Reuptake is the most common deactivation method. Neurotransmitters are taken back into the presynaptic terminal by specialized transporters located in the cell membrane. This process “recycles” neurotransmitters, enabling them to be reused in subsequent signaling.
- For instance, after the release of serotonin, dopamine, or norepinephrine, these neurotransmitters are typically reabsorbed by transporters on the presynaptic neuron, thus terminating their action on the postsynaptic receptor.
- Uptake by Glial Cells : In some cases, glial cells (support cells in the nervous system) play a role in neurotransmitter deactivation. They may take up neurotransmitters from the synaptic cleft, where they are either degraded by enzymes or recycled back to the axon terminal for reuse. This process further helps in maintaining the proper balance of neurotransmitters in the synapse.
Conclusion
Synaptic transmission is a crucial process for neuronal communication, involving neurotransmitter release from the presynaptic neuron, binding to postsynaptic receptors, and triggering excitatory or inhibitory responses. This process enables efficient and precise signal transmission, modulating neuronal activity. The regulation of neurotransmitter deactivation, through reuptake, enzymatic degradation, diffusion, and glial uptake, ensures that signals are terminated appropriately, preventing continuous activation. These mechanisms also adapt based on activity levels, supporting learning and memory. Overall, synaptic transmission is vital for all neural functions, facilitating basic and complex behaviors while maintaining the brain’s adaptability to experiences and environmental changes.
Read more on Neuropsychology
References
- Cotman, C. W., & McGaugh, J. L. (1980). Synaptic transmission. In Behavioral Neuroscience (pp. 151–208). https://doi.org/10.1016/b978-0-12-191650-3.50010-6
- Gabbiani, F., & Cox, S. J. (2010). Synaptic transmission and quantal release. In Elsevier eBooks (pp. 175–191). https://doi.org/10.1016/b978-0-12-374882-9.00012-5
- Kalat, J. W. (2019). Biological psychology. Cengage.
- Kolb, B., & Whishaw, I. Q. (2019). An introduction to brain and behavior.
- Pinel, J. (2023). Biopsychology 10th Edition. Pearson.
Dr. Balaji Niwlikar. (2025, January 20). 4 Steps of Synaptic Transmission : Communication between neurons. Careershodh. https://www.careershodh.com/synaptic-transmission/