Introduction
Long-term potentiation (LTP) is a fundamental process in neurobiology, describing a long-lasting enhancement in synaptic strength following high-frequency stimulation of neurons. First observed by Bliss and Lømo in 1973, LTP is considered a key cellular mechanism underlying learning and memory. It primarily occurs in the hippocampus but has been identified in other brain regions associated with cognitive function.
Properties of LTP
LTP exhibits three core properties that contribute to its role in synaptic plasticity-
- Specificity- Only synapses that are actively stimulated are strengthened, preventing unnecessary synaptic modifications (Ferando, Faas, & Mody, 2016).
- Cooperativity- Simultaneous stimulation by multiple presynaptic inputs enhances LTP more effectively than a single input, supporting the idea of collective learning.
- Associativity- Weak synaptic inputs, when paired with strong inputs, become potentiated, resembling classical conditioning mechanisms (Kerchner & Nicoll, 2008).
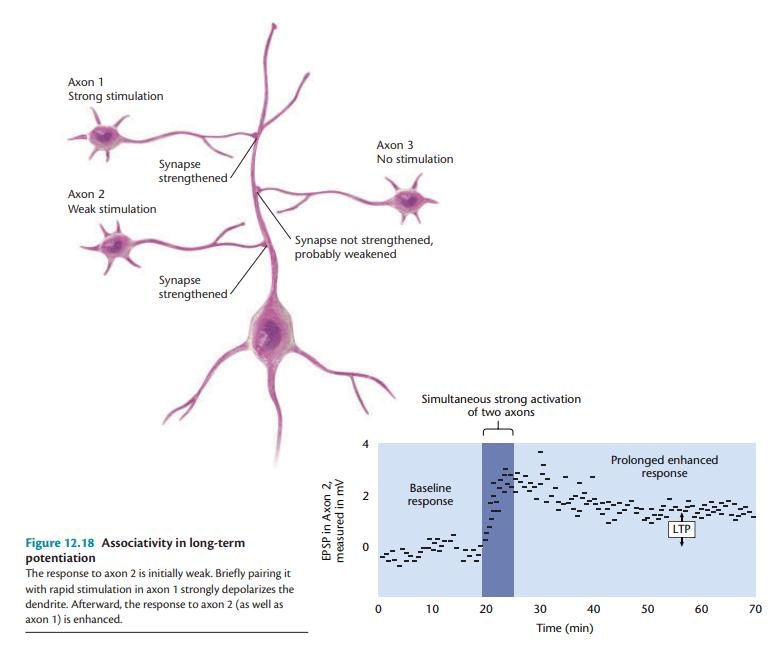
Associativity
These properties ensure that LTP is not a random process but one that selectively strengthens relevant synaptic connections, a crucial feature for efficient learning and memory encoding.
Read More- Forgetting and Its Theories
Molecular Mechanisms of LTP
There are 3 stages of LTP, there include-
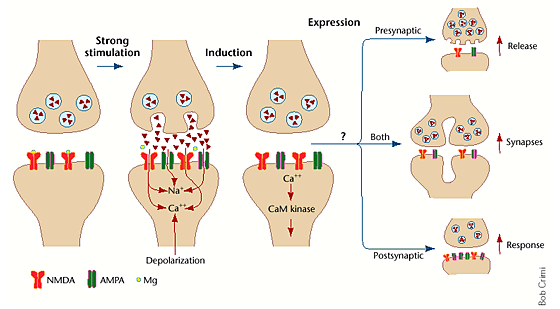
Stages of LTP
Stage 1: Induction
The initiation of LTP begins with glutamate release from the presynaptic neuron, which binds to AMPA and NMDA receptors on the postsynaptic membrane:
- AMPA receptors allow sodium (Na+) influx, generating an excitatory postsynaptic potential.
- NMDA receptors remain blocked by magnesium (Mg2+) ions under resting conditions.
High-frequency stimulation causes a strong depolarization, expelling Mg2+ from NMDA receptors, allowing calcium (Ca2+) to enter the neuron. This calcium influx is the critical trigger for synaptic strengthening.
AMPA and NMDA Receptors
AMPA and NMDA receptors are both glutamate receptors that play essential roles in synaptic transmission and plasticity. AMPA receptors mediate fast excitatory transmission by allowing Na+ ions to enter the postsynaptic neuron when activated by glutamate. NMDA receptors, on the other hand, require both ligand binding and membrane depolarization to remove the Mg2+ block, permitting Ca2+ influx. This calcium entry triggers intracellular signaling pathways crucial for LTP induction and synaptic strengthening (Kalat, 2019).
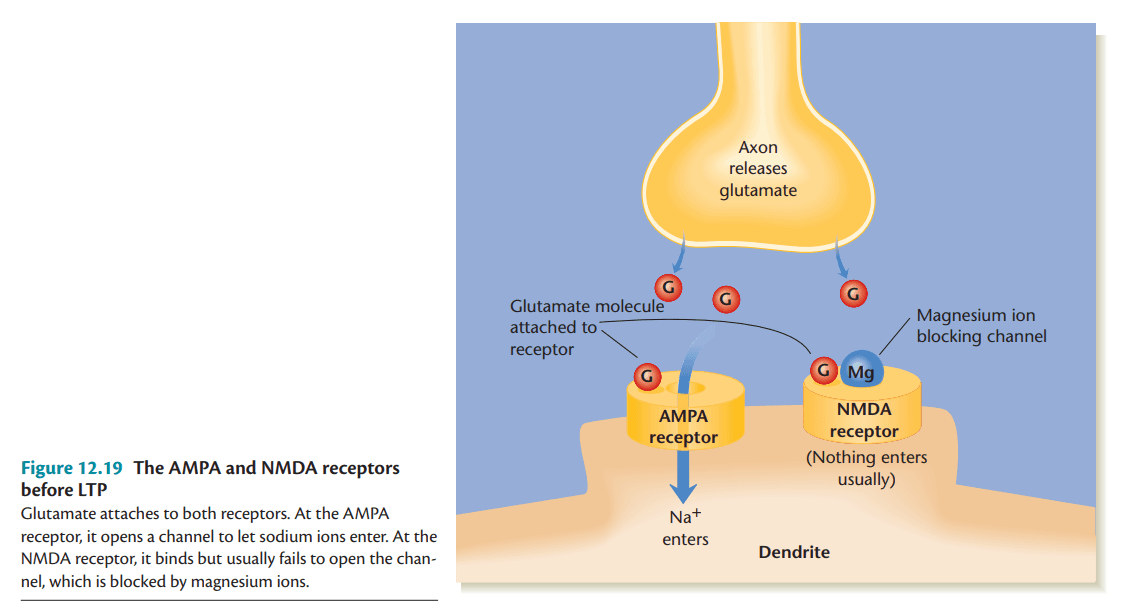
Receptors Before LTP
Stage 2: Early LTP (E-LTP)
Following NMDA receptor activation, Ca2+ influx activates several signaling molecules, including:
- CaMKII (Calcium/Calmodulin-dependent protein kinase II)- Increases AMPA receptor sensitivity and promotes their insertion into the postsynaptic membrane (Lisman, Schulman, & Cline, 2002).
- PKC (Protein Kinase C)- Facilitates further phosphorylation of AMPA receptors, enhancing synaptic response.
This phase lasts for a few hours and does not require new protein synthesis.
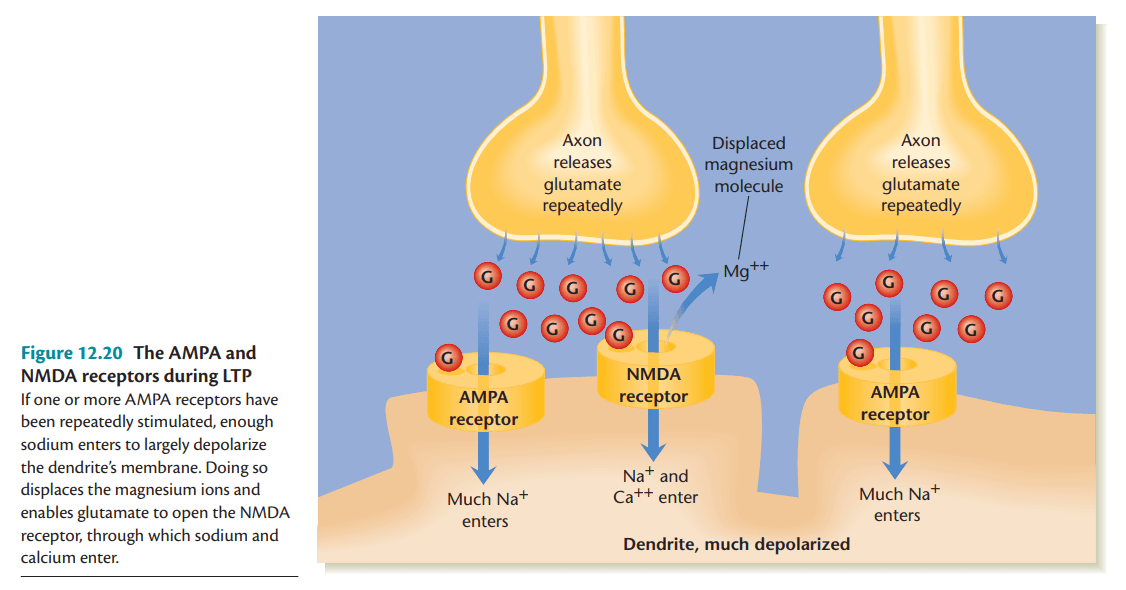
Receptors During LTP
Stage 3: Late LTP (L-LTP)
For LTP to persist beyond a few hours, changes at the genetic and structural levels occur:
- CREB (Cyclic AMP Response Element-Binding Protein) is activated, leading to gene transcription and synthesis of proteins essential for synaptic modifications.
- BDNF (Brain-Derived Neurotrophic Factor) plays a role in dendritic spine growth, increasing synaptic connectivity.
At this stage, new synapses may be formed, consolidating long-term memory storage.
Functional Significance of LTP
Some of the functional implications of LTP include-
LTP and Memory Encoding
LTP is crucial in transforming transient experiences into stable memories. Studies show that blocking NMDA receptors impairs spatial learning in rodents, indicating that LTP is necessary for hippocampal-dependent memory formation. Furthermore, imaging studies in humans suggest that learning-related synaptic changes occur in the hippocampus through LTP-like mechanisms.
LTP and Synaptic Plasticity
Synaptic plasticity, the ability of synapses to strengthen or weaken over time, underlies learning and memory. While LTP strengthens synapses, its counterpart, long-term depression (LTD), weakens synapses to prevent excessive neuronal activity. The balance between LTP and LTD is essential for cognitive flexibility, ensuring that learning remains adaptable and efficient.
Neurological Disorders
LTP dysfunction has been implicated in various neurological conditions-
- Alzheimer’s Disease (AD)- Reduced LTP is observed in AD models due to amyloid-beta accumulation, which disrupts synaptic transmission and leads to memory deficits.
- Epilepsy- Excessive LTP can contribute to hyperexcitable neuronal circuits, leading to seizures.
- Schizophrenia- Impairments in NMDA receptor function are linked to synaptic deficits, affecting cognitive processes like working memory and learning.
Therapeutic Applications
Understanding LTP has led to potential therapeutic interventions-
- Cognitive Enhancers- Drugs targeting NMDA and AMPA receptors, such as memantine, are being explored for memory enhancement in dementia.
- Brain Stimulation Therapies- Techniques like transcranial magnetic stimulation (TMS) can modulate synaptic plasticity and improve cognitive function in depression and neurodegenerative diseases.
Conclusion
LTP remains a cornerstone of neuroscience, providing insights into the cellular processes underlying learning and memory. Its intricate molecular mechanisms, role in cognitive function, and implications for neurological disorders make it a critical area of study. As research progresses, harnessing LTP-related mechanisms may lead to groundbreaking treatments for cognitive decline and enhanced learning strategies.
References
Bliss, T. V. P., & Lømo, T. (1973). Long-lasting potentiation of synaptic transmission in the dentate area of the anaesthetized rabbit following stimulation of the perforant path. Journal of Physiology, 232(2), 331–356.
Ferando, I., Faas, G. C., & Mody, I. (2016). Altered gamma oscillations during physiological conditions and epileptic seizures. Nature Communications, 7, 11388.
Kalat, J. W. (2019). Biological Psychology (13th ed.). Cengage Learning.
Kerchner, G. A., & Nicoll, R. A. (2008). Silent synapses and the emergence of a postsynaptic mechanism for LTP. Nature Reviews Neuroscience, 9(11), 813–825.
Lisman, J., Schulman, H., & Cline, H. (2002). The molecular basis of CaMKII function in synaptic and behavioural memory. Nature Reviews Neuroscience, 3(3), 175–190.
Zakharenko, S. S., Zablow, L., & Siegelbaum, S. A. (2001). Visualization of changes in presynaptic function during long-term synaptic plasticity. Nature Neuroscience, 4(7), 711–717.
Subscribe to Careershodh
Get the latest updates and insights.
Join 13,999 other subscribers!
Niwlikar, B. A. (2025, February 2). Long-Term Potentiation and Its 3 Important Stages. Careershodh. https://www.careershodh.com/long-term-potentiation/