Cognitive Map
A cognitive map is a mental representation of spatial information that helps us navigate and understand our environment. This concept extends beyond simple visual images; it encompasses the knowledge of spatial relationships and the layout of our surroundings. Cognitive maps are integral to how we perceive and organize geographic information, allowing us to navigate through both familiar and unfamiliar spaces efficiently.
Cognitive maps are used in various contexts, from finding your way around a new city to understanding the layout of a building or campus. They are not merely visual but involve a complex integration of sensory input, memory, and spatial reasoning. For example, when you learn the route to a new location, you create a cognitive map that includes the relationships between landmarks, distances, and directions. This mental representation helps you plan and execute your navigation tasks.
Research on cognitive maps focuses on how we form, store, and utilize these spatial representations. It examines how cognitive maps reflect reality and how they can sometimes lead to systematic errors. These errors often arise due to cognitive heuristics—simplified strategies that help us make judgments and decisions but can sometimes lead to inaccuracies. Understanding cognitive maps involves studying how we perceive distances, shapes, and relative positions in our environment and how these perceptions are influenced by our mental processes and experiences.
Classical Experiment on Cognitive Maps: Tolman and Honzik’s Rat Experiment
In the realm of psychology, one of the most influential studies on cognitive maps was conducted by Edward C. Tolman and C.H. Honzik in the 1940s. Their experiment provided critical insights into the nature of learning and memory, challenging the behaviorist view prevalent at the time. This study not only broadened our understanding of how animals navigate and learn but also introduced the concept of cognitive maps—internal mental representations of the environment.
The Theoretical Background
Before Tolman and Honzik’s research, behaviorism was the dominant paradigm in psychology, emphasizing that animal behavior could be fully explained by simple stimulus-response (S-R) associations. According to this view, learning was thought to involve the direct reinforcement of specific responses to stimuli. Tolman, however, proposed a different perspective. He suggested that organisms, including humans and animals, create internal representations of their environment, which he termed cognitive maps. These maps are mental constructs that help organisms navigate and understand their surroundings beyond mere S-R associations.
The Experimental Set-Up
Tolman and Honzik’s experiment involved a meticulously designed maze to explore how rats learn and navigate their environment. The maze consisted of a complex network of paths with various possible routes leading to a goal box that contained a food reward. The experimental design included three distinct groups of rats, each subjected to different training conditions:
1. Group 1 (Consistent Reward Group): Rats in this group were rewarded with food every time they successfully reached the goal box. This group was meant to reinforce the idea that repeated reinforcement would lead to learning the maze through direct rewards.
2. Group 2 (Delayed Reward Group): Rats in this group were not rewarded during the first ten days of training. After the initial period, they were then given rewards upon reaching the goal box. This group was designed to test whether the lack of immediate rewards would hinder learning or if the rats could still form cognitive maps.
3. Group 3 (No Reward Group): Rats in this group did not receive any rewards throughout the entire experiment. This condition was included to observe if the lack of reinforcement affected the rats’ ability to learn and navigate the maze.
Training Phase
During the training phase, all groups of rats were initially placed at the starting point of the maze. For Group 1, each successful navigation of the maze was immediately reinforced with a food reward. Group 2 received no reinforcement initially, which allowed researchers to test the effects of delayed rewards on learning. Group 3, lacking any reinforcement, served as a control to examine whether rewards were necessary for maze navigation.
Over several trials, the rats in Group 1 learned to navigate the maze efficiently, following the path that led directly to the food. The consistent reward reinforced their learning and made them adept at the maze layout.
Test Phase
After the initial training phase, Tolman and Honzik introduced changes to the maze to assess how well the rats had learned and adapted. For example, they blocked one of the previously learned paths and opened a new route to the goal box. This change was designed to test whether the rats had developed a cognitive map of the maze layout or were simply relying on memorized responses.
Tolman observed how the rats adapted to the new configuration of the maze. Rats that had been previously trained in Group 1 continued to navigate the maze effectively, taking the new route to the goal box without difficulty. Rats from Group 2, who had been rewarded only after ten days of training, also demonstrated significant adaptability. Even though they had been deprived of immediate rewards during the early training period, they quickly adapted to the new maze layout once they began receiving rewards. Group 3 rats, who had never received any rewards, showed limited navigation improvement, but they still managed to find the goal box more effectively than would be expected by chance alone.
Findings and Implications
Tolman and Honzik’s findings were groundbreaking. The rats demonstrated an ability to adapt to changes in the maze by finding alternative routes to the goal box, indicating that they had developed an internal representation of the maze—a cognitive map. This ability to navigate the maze even after changes in the layout suggested that learning involved more than just a series of conditioned responses to stimuli.
Their research provided compelling evidence that cognitive maps play a crucial role in navigation and problem-solving. It showed that animals, including humans, utilize these internal maps to understand and adapt to their environment, challenging the behaviorist view that behavior is solely a product of external rewards and stimuli. This experiment laid the foundation for further research in cognitive psychology, influencing how we understand spatial learning, memory, and cognitive processes in both animals and humans.
Tolman and Honzik’s work remains a cornerstone in the study of cognitive maps, underscoring the complexity of learning and the sophisticated mental processes that underpin our interactions with the world around us.
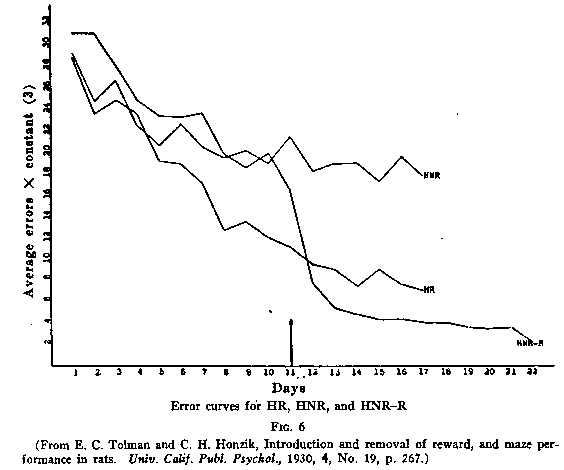
Cognitive Map (average error and days)
Implications
Tolman’s experiment provided compelling evidence for the existence of cognitive maps. His findings suggested that rats were not merely learning specific responses to stimuli but were instead forming mental maps of the maze that allowed them to navigate flexibly. This was a significant departure from the behaviorist perspective and supported the idea that animals, including humans, use internal cognitive representations to understand and interact with their environments.
The experiment demonstrated that cognitive maps play a crucial role in navigation and spatial understanding. His research laid the groundwork for further studies on spatial cognition and contributed to the broader field of cognitive psychology by highlighting the importance of internal mental representations in guiding behavior.
Factors that Influence Cognitive Maps
Distance
They are subject to various distortions influenced by factors such as the number of intervening landmarks, category membership, and the presence of landmarks.
- Number of Intervening Cities
One significant factor affecting distance estimates in cognitive maps is the number of intervening cities or landmarks between two locations. Thorndyke’s (1981) classic study explored this effect by using a hypothetical map with cities distributed across various routes. Participants were trained to accurately reconstruct the map and then asked to estimate distances between pairs of cities.
The results revealed that the number of intervening cities significantly influenced distance estimates. When cities were separated by no intervening cities, participants estimated the distance to be shorter than when multiple cities were in between. For instance, a distance of 300 miles between two cities was estimated as 280 miles when no other cities were intervening but as 350 miles when three cities were present. This pattern of errors aligns with heuristic reasoning, where people perceive distances as shorter when fewer landmarks are encountered and longer when more landmarks are present.
- Category Membership
Category membership also impacts how we estimate distances. Hirtle and Mascolo (1986) found that people tend to estimate distances differently based on the category to which locations belong. In their study, participants estimated distances between pairs of locations on a map after the map was removed.
The results showed that people often shifted locations closer together if they belonged to the same category (e.g., government buildings). This effect, known as the same-category heuristic, also extends to larger-scale distances. For example, Friedman and colleagues found that students estimated distances between cities in different countries as greater than between cities within the same country, despite the actual difference being much smaller. This bias is termed border bias, where distances across geographic or administrative boundaries are perceived as larger than those within the same boundary.
- Landmarks
Landmarks play a crucial role in shaping our cognitive maps and distance estimates. The landmark effect, as demonstrated by McNamara and Diwadkar (1997), shows that distances are often perceived as shorter when traveling towards a landmark compared to traveling away from it. Participants estimated shorter distances between landmarks and non-landmarks when moving towards the landmark than when moving away. This effect illustrates how prominent geographical features can influence our perception of distance and highlights the importance of context in spatial judgments.
Shape
Cognitive maps also represent shapes, and these representations can be distorted. Moar and Bower’s (1983) study on cognitive maps of Cambridge, England, revealed that people tend to regularize angles in their mental maps. Participants consistently estimated angles formed by intersecting streets as closer to 90 degrees than they actually were. For example, angles that were actually 67, 63, and 50 degrees were estimated as 84, 78, and 88 degrees, respectively. This distortion is attributed to the 90-degree-angle heuristic, where people mentally simplify the shapes of intersections to conform to a common, regular pattern. This tendency to regularize angles helps in storing and recalling spatial information but can lead to systematic biases in distance and shape representation.
These studies collectively illustrate how cognitive maps are influenced by various cognitive biases and heuristics. They show that while cognitive maps are useful for navigation and spatial understanding, they are shaped by our mental processes and can lead to systematic distortions in how we perceive distances and shapes. Understanding these effects is crucial for improving our spatial cognition and developing more accurate navigational tools.
Relative Position
Cognitive maps, or mental representations of spatial information, often exhibit systematic biases due to heuristics we use to simplify complex geographic information. Two notable heuristics affecting our perception of relative positions are the rotation heuristic and the alignment heuristic. These heuristics can lead to distortions in our cognitive maps, causing us to misjudge the relative positions of geographic locations.
- The Rotation Heuristic
The rotation heuristic involves mentally rotating geographic structures to align them with more familiar orientations. When we encounter a slightly tilted structure, such as a coastline or a border, we tend to remember it as being more vertical or horizontal than it actually is.
For example- In the case of San Diego and Reno, the coastline of California is slanted in reality. However, due to the rotation heuristic, people might mentally rotate this coastline to make it appear more vertical. As a result, they might incorrectly conclude that San Diego, which is geographically east of Reno, is farther west. Similarly, people might misjudge the relative positions of Detroit and Windsor, with Windsor actually being south of Detroit but perceived as being north due to this heuristic.
Tversky (1981) demonstrated this effect with the San Francisco Bay Area. Most participants showed evidence of the rotation heuristic, perceiving the California coastline as more north-south oriented than it actually is. This distortion has been observed in various cultures, including Israel, Japan, and Italy (Glicksohn, 1994; Tversky et al., 1999).
2. The Alignment Heuristic
The alignment heuristic involves remembering a series of geographic structures as being more aligned or lined up than they actually are. This heuristic leads us to align separate locations or regions in a straight line, even when they are not.
For example- Consider the relative positions of Rome and Philadelphia. In reality, Rome is north of Philadelphia, but the alignment heuristic might lead people to perceive them as aligned along the same latitude because of the tendency to align Europe and North America in a straight line. This heuristic can result in incorrect judgments about the relative north-south positions of these cities.
Tversky (1981) found that many students misjudged the relative positions of cities, with a majority incorrectly believing Philadelphia was north of Rome. This heuristic has been confirmed in other studies, particularly in comparisons between northern cities in North America and southern cities in Europe (Friedman et al., 2002).
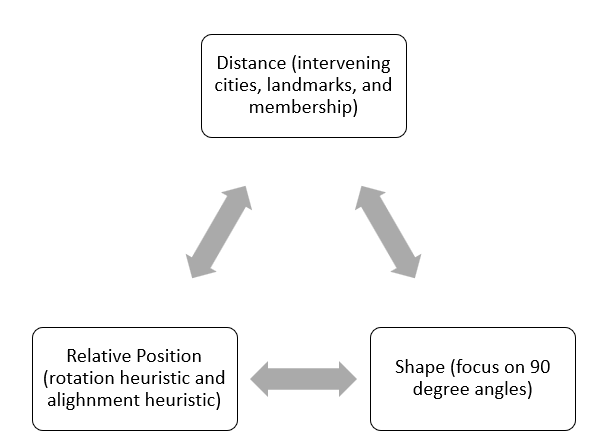
Factors Influencing Cognitive Maps
Conclusion
The study of mental imagery reveals its profound impact on cognitive processes and practical applications. Visual imagery, with its roots in classical research and contemporary findings, underscores how mental representations mirror perceptual experiences. The debate between analog and propositional codes continues to shape our understanding of how mental images are stored and manipulated. Research into auditory imagery, though less extensive, demonstrates its potential and the need for further exploration. Together, these insights contribute to a broader understanding of how mental imagery functions and its implications for both everyday life and specialized practices, such as rehabilitation and cognitive training.
References
Friedman, A., & Waller, D. (2002). The alignment heuristic in geographical judgments: The case of the American Midwest. Journal of Experimental Psychology: Applied, 8(3), 215–227.
Galotti, K. M. (2018). Cognitive psychology in and out of the laboratory. Thomson Brooks/Cole Publishing Co.
Hirtle, S. C., & Mascolo, M. F. (1986). Cognitive maps and spatial memory: An overview. International Journal of Psychology, 21(2), 165–186.
Matlin, M. W., & Farmer, J. A. (2016). Cognitive psychology. John Wiley & Sons.
McNamara, T. P., & Diwadkar, V. A. (1997). Cognitive maps and the landmark effect. Journal of Experimental Psychology: Learning, Memory, and Cognition, 23(1), 1–16.
Thorndyke, P. W. (1981). The role of landmarks in predicting spatial relations. Cognitive Psychology, 13(1), 138–149.
Tolman, E. C. (1948). Cognitive maps in rats and men. Psychological Review, 55(4), 189–208.
Tversky, B. (1981). Distortions in cognitive maps. Geoforum, 12(2), 211–217.
Dr. Balaji Niwlikar. (2024, September 10). Cognitive Map- 3 Powerful Factors that Influence them!. Careershodh. https://www.careershodh.com/cognitive-map/